High Energy Physics
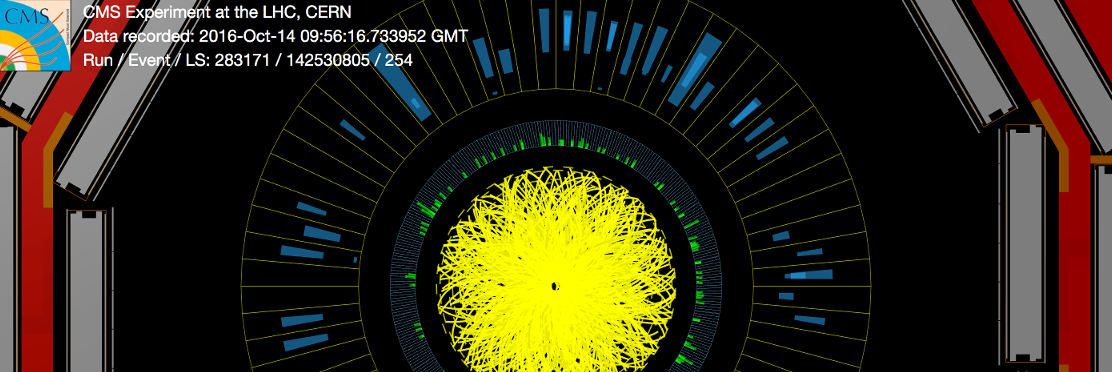
© 2016 CERN, for the benefit of the CMS Collaboration – Collisions recorded by the CMS detector on 14 Oct 2016 during the high pile-up fill
SPRACE’s fundamental research team is dedicated to high energy physics (HEP), working in the CMS experiment of the Large Hadrom Collider (LHC). Our main research lines include the search for new physics beyond the standard model, particularly the search for dark matter candidates and the study of the strong interacting matter through the collision of relativistic heavy ions.
HEP is an essential component of the physical sciences, focused on the study of the nature of matter and energy, their fundamental building blocks and interactions. Since Rutherford’s breakthrough experiment a century ago, the investigation in HEP is accomplished through high energy particle accelerators, which have been able to unravel the deep constituents of matter, explore their behavior at short distances and produce new heavy particles that are not part of the usual matter that makes up the everyday world.
The discovery of the Higgs boson by CERN’s Large Hadron Collider (LHC), announced on 4 July 2012, provided an important ratification of the Standard Model (SM). Proposed in the late 1960s to describe the electroweak interaction, the SM has survived an intense experimental scrutiny during the last four decades. Maybe the most remarkable results were obtained by the Large Electron-Positron collider, which in the 1990s provided compelling evidence in favor of the SM by making precise measurements of several parameters predicted by the theory. On the other hand, the theoretical research in the field kept on developing new ideas that needed a high-energy facility to be tested. The LHC was able to finally provide this environment capable of exploring the TeV frontier.
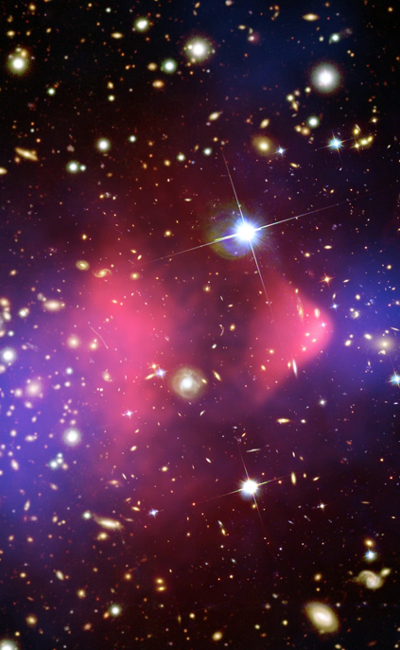
NASA/CXC/CfA/ M.Markevitch et al.: The Matter of the Bullet Cluster
Beyond the Standard Model
Despite the impressive successes of the Standard Model, our understanding of the fundamental interactions is far from being complete.
Cosmological observations, for example, show that the speed at which stars revolve around the center of their galaxies is different from the speed predicted by theory, which takes into account all the matter we can see. Scientists explain this discrepancy by proposing the existence of matter that we cannot see – dark matter. This type of matter would make up about 23% of the universe, while the visible matter would account for only 4%. Despite being much more abundant than ordinary matter, we still do not know what dark matter is made of. Research conducted in the LHC looks for new particles that may explain the nature of this mysterious substance.
If we add up visible matter and dark matter, we would have only 27% of the constitution of the universe. It is currently believed that the remaining 73% is dark energy – the energy responsible for the acceleration of the expansion of the universe. The nature of dark energy is also still not fully understood.
Another unresolved question in HEP is: why is there more matter than antimatter in the universe? This problem, called baryonic asymmetry, derives from the fact that the Big Bang, in principle, should have created equal amounts of matter and antimatter. When we analyze the universe today, however, we see a far greater amount of matter. Experiments at the LHC may also shed light on this issue where the interplay between particle physics and cosmology exists.
Heavy Ion Physics
In the strong interaction sector of the SM, the challenge is to understand the properties of hadronic matter: the matter that comprises almost the entire visible universe, from protons and neutrons to extremely dense stellar objects. The basic building blocks of hadronic matter at its most fundamental level are quarks and gluons, which are described by the theory of Quantum Chromodynamics (QCD). Quarks and gluons are not observed in isolation; they are bound inside the hadrons, as consequence of a property of QCD known as confinement. Understanding the confinement of quarks and gluons is one of the greatest challenges of contemporary physics.
Asymptotic freedom is another prominent property of QCD: the strength of the interactions among quarks and gluons decreases when they come close together. As a consequence, it was suggested that matter at densities much higher than the density of normal nuclei consists of a soup of freely moving quarks and gluons, the so-called quark-gluon plasma (QGP). Such a state of matter is conjectured to have existed in the very early Universe and the best way to recreate QGP in the laboratory is through high energy collisions of heavy ions (HI), large nuclei of atoms stripped of their electron content. High energy HI collisions can produce systems where large amounts of energy are concentrated in a small volume, where we could expect to recreate small samples of this primordial matter.
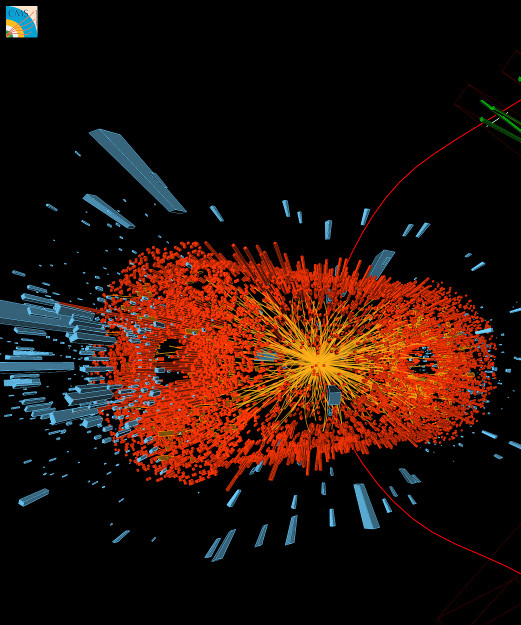